Ep 161: Evan Sengbusch - President, Phoenix
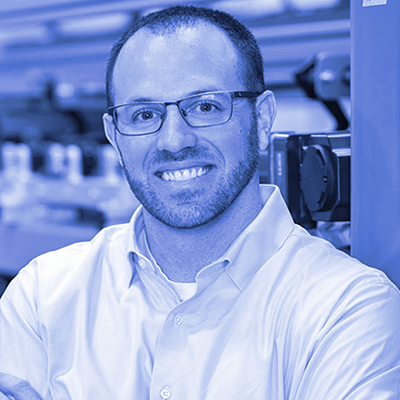
Show notes
Q1 - Applications of Medical Physics
Naomi Senehi: How did you get into the nuclear field?
Evan Sengbusch: Evan Sengbusch grew up in Ames, Iowa, and studied physics and math at the University of Iowa. He was interested in experimental particle physics and spent some time as an undergraduate at CERN in Switzerland working on the collider. Through a research opportunity on campus, Sengbusch got involved in medical physics at the Positron Emission Tomography Imaging Center, which uses radioisotopes for medical imaging. In his early years of studying physics, Sengbusch focused on theoretical physics, but began to explore more applied applications doing experimental plasma physics before spending time at CERN. He appreciated the immediate impact of his work on the patients coming through on a daily basis, utilizing new isotopes that he worked to develop. Evan Sengbusch completed his PhD in medical physics at the University of Wisconsin and become connected with his advisor “Rock” Mackie, well known in the field and a serial entrepreneur. Mackie started a company called TomoTherapy, which put a linear accelerator on a gantry and treat patients in a helical fashion for medical therapy. Sengbusch discovered that he liked the fast pace of industry. His PhD defense was on compact proton therapy systems for cancer treatment, which was directly related to his work with CPAC (Compact Particle Accelerator Corporation).
Q2 - Proton Versus Photon Therapy
Naomi Senehi: What problem were you trying to solve with your research in medical physics and what challenges did you face?
Evan Sengbusch: Evan Sengbusch’s doctoral research was focused on compact proton therapy systems for cancer treatment. Currently technology for cancer treatment includes chemotherapy chemicals or external beam radiation therapy, which shoots high energy x-rays into a body targeted to kill cancer cells. An alternative treatment is proton therapy; protons are heavy particles whose depth can be controlled by the energy in the particle. Photons go all the way through the body and damage healthy tissue behind the cancer. It is difficult to accelerate protons to appropriate beam energies and currents for treatment. Sengbusch’s research focused on how to bring the size and cost of the equipment down to be more manageable practical so more people can get access to this treatment. Beam current, treatment angle, and gantry angle is started to become an automated optimization problem that can bring cost down. Evan Sengbusch started working with Phoenix right out of graduate school in 2012. Phoenix makes lower energy, higher current deuteron accelerators. Deuterium is an isotope of hydrogen. Deuterons are accelerated into deuterium and tritium targets to generate nuclear fusion, producing neutrons. Challenges include creating neutron sources that are strong enough to provide the right energy spectrum and flux to the target and developing targets that are specific enough to target only cancer cells and not normal tissue.
Q3 - Medical Radioisotope Production
Naomi Senehi: How does the number of treatments that you need with the NCT compared to proton therapy and photon therapy?
Evan Sengbusch: There is an opportunity to do a smaller number of fractions for different cancer therapies. Each treatment is a fraction to get up to a total accumulated dose in order to prevent acute side effects to the normal tissue if it were exposed to all the radiation at once. Hypofractionation, a smaller number of fractions, is being explored with photon and proton therapy. Phoenix received an award from the Department of Energy (DOE) in 2009 to develop a domestic supply of the medical radioisotope Molybdenum 99 (Moly-99), which is used in medical imaging procedures such as single-photon emission computed tomography (SPECT) and cardiac stress tests. Moly-99 is only made at nuclear reactors and there is a limited number of reactors designed appropriately to make it, all outside the Western hemisphere. Phoenix developed a proposal to use an accelerator-driven neutron source with a low-enriched uranium subcritical assembly around it. Nuclear fission is induced and one of the byproducts is Moly-99. In 2010, Phoenix started SHINE Medical Technologies which is currently building a production facility dedicated to producing Moly-99 with this technique. Commercial production of isotopes is expected in 2021 and to produce half of the world’s demand of Moly-99.
Q4 - Neutrons as a Visualization Tool
Naomi Senehi: Why did Phoenix break off into SHINE to complete Moly-99 production as a partner company?
Evan Sengbusch: Phoenix has grown outside of the medical industry and takes its products to the Department of Defense (DOD) and the nuclear energy industry. Phoenix’s founder needed private investment to get the high volume medical radioisotope production and started SHINE to manage this portion of work. One of Phoenix’s core innovations was utilizing a gaseous as opposed to a solid target. Approximately ten times the number of neutrons are produced with a gaseous target. The system has a large differential pumping section which creates a billion times pressure differential between the target regime, where neutrons are generated, and the accelerator side, where the deuterium ion beam is accelerated to induce the reaction. For the DOD, Phoenix is developing systems that use neutrons as an inspection tool as they pass through an industrial component to provide visualization. Neutrons are good at penetrating high density material to visualize low density material, which is different that how x-rays function. This could be used to find defects inside energetic systems.
Q5 - Accelerator-based Imaging Systems
Naomi Senehi: What’s the cost difference of x-ray versus accelerator-based system?
Evan Sengbusch: Accelerating ions is harder to accelerate electrons as done with x-rays, so neutron systems are more expensive today than x-ray systems. X-ray inspection systems have also been built for decades, whereas neutron systems are fairly early in development. SHINE has signed supply agreements with a number of customers who take Moly-99 and put them into a generator, which allows Moly-99 to decay into Technetium, the useful isotope that goes into the patient. General Electric looked at modifying a power reactor to product Moly-99, but the modifications required to get access to the core was not economical. Due to this, only research reactors are used for production.
Q6 - Neutron-based Inspection of Nuclear Fuel Rods
Naomi Senehi: What is it like being pro-nuclear but competing head on with nuclear for radioisotope production?
Evan Sengbusch: Evan Sengbusch does not see Phoenix technology as a replacement for nuclear reactors. The technology is not aimed at producing energy at this time, but Phoenix does build neutron-based inspection systems that measure enrichment of nuclear fuel before it goes to refuel power generating reactors. Each pellet is designed to have a certain enrichment along a reactor’s profile, based on neutronic and heat distribution. Incorrect levels of enrichment in fuel rods could cause a localized hot spot, affect distribution of neutrons, or cause a cracked fuel rod that becomes a big clean-up problem. This enriched fuel is radiated with a high flux of thermal neutrons that induce nuclear fission; the radiation coming off the fuel rod is measured to determine the enrichment of the fuel rod. This process used to be performed with Californium, which is increasingly difficult to create. Electronic neutron sources are now being used instead of radioisotopes in some cases.
Q7 - How Neutrons Can Detect Flaws in Turbine Blades
Naomi Senehi: What’s in the future for Phoenix?
Evan Sengbusch: Phoenix is scaling up to build the first batch of eight accelerators for the SHINE project to come online in 2021. The company is also working closely with the Army focused on imaging and has a new facility called the Phoenix Neutron Imaging Center coming online in Madison, WI this summer. This high output neutron source will have two different imaging facilities tied to it. One facility is for thermal neutron imaging, which could be used to inspect turbine blades for residual ceramic in the turbine cooling channel. These turbine blades are currently inspected at research reactors, which is a large interruptor to the manufacturing process. The other imaging facility has a fast neutron imaging capability. Low energy neutrons are desired for smaller components because they have a higher chance of finding the ceramic material being searched for, but they have limited penetration. Sengbusch sees the biggest challenge as convincing the market to adopt new technologies.
Q8 - Future of Nuclear Fusion Energy
Naomi Senehi: What do you hope for the future of nuclear technology?
Evan Sengbusch: The original vision of Phoenix was to commercialize near term applications of nuclear fusion technology as a way to generate incremental revenue to reinvest in taking the technology to the next level with a long term goal of nuclear fusion energy. All the work Phoenix does will benefit future approaches for using nuclear fusion as a clean energy source.
7-10 Bullets
- How Evan Sengbusch’s background in particle physics led to a career in medical physics - Production of lower energy, higher current deuteron accelerators as neutron sources - Why a domestic supply of Molybdenum 99 is vital to the nation’s medical industry - How neutrons are used as an inspection tool for complex, high density materials and components - Role of research reactors in medical radioisotope production - Neutron-based inspection systems for enrichment in nuclear fuel rods - How the President of Phoenix, Evan Sengbusch, is commercializing near term applications of nuclear fusion technology.
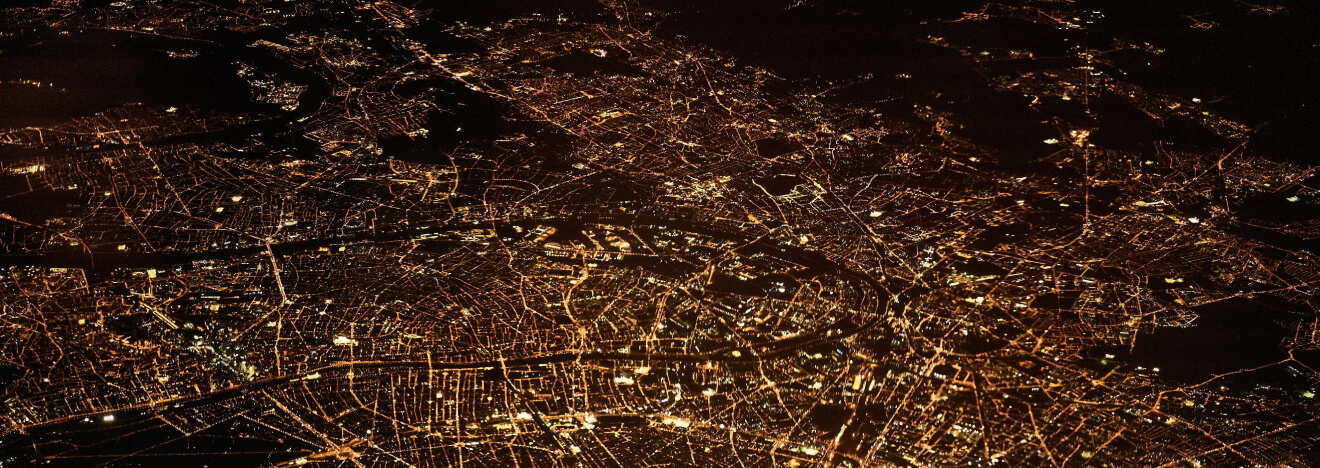